The LSM 700 laser scanning confocal microscope from Carl Zeiss is designed for ease of use when investigating complex phenomena. The instrument can detect up to four color signals at frame rates approaching 5 frames per second at 512 x 512 pixels. Efficient separation of the fluorescence signals by selective laser excitation coupled to efficient splitting of the emission using the variable secondary dichroic (VSD) beamsplitter prevents crosstalk and enables spectral imaging as well as linear unmixing of highly overlapping fluorophores. Among the advanced features of the VSD beamsplitter is that all portions of the emission spectrum are utilized for determining each spectral data point.
The tutorial initializes with a labeled cut-away diagram of the ZEISS LSM 700 scanning module appearing in the window. Light from the microscope passing through the pinhole is directed onto the surface of the variable secondary dichroic (VSD) mirror. A portion of that light is then transmitted to one of the photomultipliers, while another portion is reflected into a second photomultiplier. The tutorial labels can be toggled on or off using the Labels checkboxes. The position of the VSD mirror can be altered by using the Beam Splitter Position slider.
Perhaps the simplest configuration using a laser scanning confocal microscope for spectral imaging couples a diffraction grating with an adjustable slit that can be mechanically translated across the input window of the photomultiplier detector. Fluorescence emission passing through the pinhole is reflected onto the diffraction grating surface using a dichromatic mirror. The spectrally separated light is then projected through the slit and into the photomultiplier. The spectral bandwidth can be modulated by increasing or decreasing the slit size and the wavelength region presented to the photomultiplier is determined by the position of the slit in relation to the dispersed light from the grating. Another innovative, yet simple, design incorporates an interference filter device termed a variable secondary dichroic (VSD), which serves as a beamsplitter to direct selected wavelength bands into separate photomultiplier detectors (see Figure 1). The vertical position of the VSD unit with respect to the emission beam in the confocal optical train determines the wavelength band that is transmitted to the first of two photomultipliers. The second photomultiplier receives light of longer wavelength that is reflected from the surface of the VSD. Wavelength selection using the VSD is easily achieved simply by shifting the position of this dichromatic beamsplitter element.
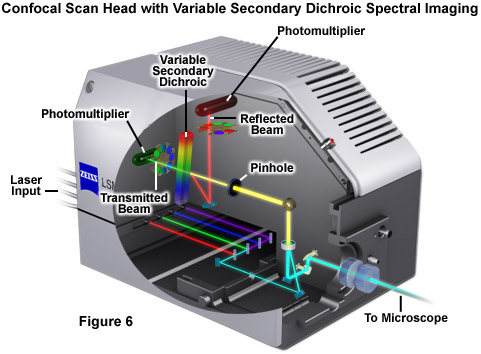
In addition to the capability of generating precise lambda stacks, the implementation of methodology for variable bandpass detection and spectral discrimination in laser scanning confocal microscopy also offers an unprecedented level of flexibility in fine-tuning emission bandwidths for general imaging that dramatically exceeds the capabilities of traditional interference filters. All too often, the fruitful separation of fluorescence emission spectra fails simply due to the fact that the instrument is not equipped with the optimum filters. Most of the spectral imaging approaches described above provide the ability to freely configure the detection wavelength range, effectively enabling the design of custom bandpass settings for virtually any fluorophore of interest. For example, using an instrument equipped with a multichannel detector, selected channels can be binned to produce an image in the desired emission range, regardless of whether the specimen is destined to ultimately be analyzed through linear unmixing algorithms. Confocal instruments having a diffraction grating and slit-detector system can easily be adjusted to gather emission across bandpass regions several hundred nanometers wide. Collectively, modern spectral imaging confocal instruments offer a tremendous advantage for eliminating fluorophore bleed-through during routine imaging of existing fluorophores, as well as the capability of easily creating custom emission bandpass configurations for the optimum detection of new probes as they are developed.
Contributing Authors
Tony B. Gines and Michael W. Davidson - National High Magnetic Field Laboratory, 1800 East Paul Dirac Dr., The Florida State University, Tallahassee, Florida, 32310.